NMR spectroscopy and CEST imaging
Revealing Cancer Metabolism In Vivo
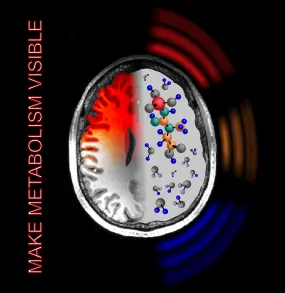
Our research group develops non-invasive metabolic and molecular MR techniques using 1H and X nuclei, comprising high-resolution NMR spectroscopy (MRS) and MR spectroscopic imaging (MRSI) at ultra-high fields (B0 ≥ 7T), chemical exchange saturation transfer (CEST) MR imaging, and hyperpolarized carbon-13 (HYP 13C). A particular clinical research focus is the molecular tissue characterization of brain tumors for the stratification of genetic subtypes and the assessment of response to therapies. Another focus is the development of novel imaging techniques for the improved molecular characterization of breast cancers.
Research Topics
- Imaging of physiological parameters, in particular pH values
- Assessment of tissues' energy metabolism
- Assessment of changes in tissues' bulk proteome
- Identification of imaging surrogates for clinical biomarkers using metabolite signals and molecular contrast mechanisms
- Development of novel molecular imaging techniques
- Clinical translation of developed imaging methods
Moreover, our research group is participating in the following consortia projects:
MIRACLE, the platform for virtual biopsies; introducing Metabolic MRI-as-a-Service for oncologic care (HORIZON-EIC funded project)
HYPERBOLIC – Visualizing Hallmarks of Cancer through Tailored Hyperpolarized Magnetic Resonance Imaging (DKTK funded project)
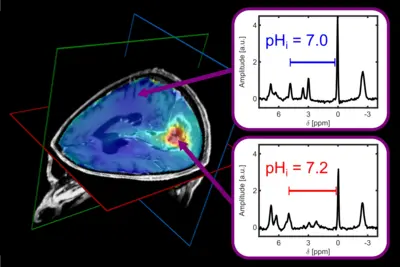
NMR spectroscopy (MRS) enables the detection of signals of atomic nuclei with spin I≠0 bound to biomolecules. The biochemical information is obtained non-invasively and without ionizing radiation.
Several spin species yield multiline in vivo NMR spectra in acquisition times of a few minutes, for example spin-½ nuclei like 1H (information about brain metabolites) and 31P (information about energy metabolism, membrane phospholipid turnover and intracellular pH). The combination of MRS with MR imaging (MRI) techniques is called MR spectroscopic imaging (MRSI), which yields maps of the regional abundance and distribution of metabolites in tissue.
The introduction of ultrahigh–field (UHF) MR scanners (magnetic field strength B0 ≥ 7 Tesla) opened new perspectives for MRS and MRSI owing to increased sensitivity and spectral resolution, enabling to detect more metabolites. To promote clinical applicability of MRSI, our research group focuses on the development of high-resolution MRSI methods at UHF overcoming several technical challenges occurring at higher fields.
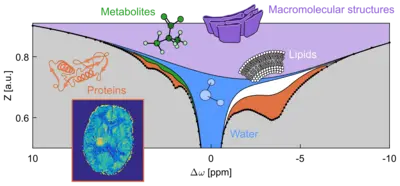
Metabolites of low molecular mass (e.g. creatine and glucose), proteins, and other macromolecular structures carry weakly bound protons (1H nuclei) at their surface which can exchange with protons in neighboring bulk water molecules. This process, named chemical exchange (CE), occurs spontaneously and depends on concentration, pH, temperature, and other properties of the solution.
Exchanging protons can resonate at different frequencies ("chemical shifts") in the 1H NMR spectrum. Hence, they can be labeled selectively (e.g. by resonant radiofrequency irradiation) to induce equal population of the two 1H spin states in a magnetic field – this technique is called saturation. Chemical exchange pumps this information into the water pool. Ongoing irradiation accumulates saturation in the water pool and produces the CEST effect (chemical exchange saturation transfer), i.e. a detectable reduction of the NMR signal of water protons. The amplification effect can make up several orders of magnitude. Note that in contrast to conventional NMR spectroscopy (MRS), which acquires the signal of tightly bound, hence non-exchanging nuclei (1H, 13C, 19F, 31P...), CEST employs the water signal to indirectly detect the signal of weakly bound protons in biomolecules.
When CEST is combined with MR imaging (MRI) techniques, different entities of cellular compounds – as illustrated in the Figure – can be scanned in living tissue (in vivo) with a sensitivity comparable to conventional MRI. Since the discovery of the phenomenon in the year 2000, CEST-MRI has been applied to diagnostic imaging of various diseases (e.g. tumors, stroke, neurodegenerative disorders). These studies revealed new information about those pathologies on a molecular level.
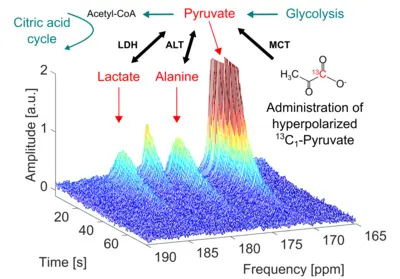
The NMR signal in living tissue is fundamentally limited by the available thermal polarization of P ≈ 10-6. Outside tissues, though, polarization levels can be manipulated to values of P ≈ 0.5 using hyperpolarization techniques, e.g. dynamic nuclear polarization (DNP), yielding a tremendous gain in NMR signal by about five orders of magnitude. Following the administration of substances carrying hyperpolarized spins, highest NMR signal is also exploitable in living tissue for the lifetime of polarized states (on the order of minutes), enabling NMR applications of unprecedented sensitivity.
One such application is metabolic MR using hyperpolarized 13C (see Figure): 13C-enriched, endogenous substrates (e.g. 1-13C-Pyruvate) are hyperpolarized and subsequently administered into living beings, where cellular metabolism 'transfers' the hyperpolarized 13C nuclei to other substances. As a consequence, the NMR signals of downstream metabolic products (e.g. 1-13C-Lactate) are also enhanced and become detectable. Utilizing dynamic 13C NMR techniques, each hyperpolarized metabolite can be identified by its individual 13C resonance frequency (chemical shift), and tracking the time course of hyperpolarized signals enables to quantify enzymatic activity (e.g. Lactate Dehydrogenase) in real time.
This way, the metabolic alterations of cancer tissues can be assessed, e.g., the increased activity of Lactate Dehydrogenase leading to an increased flux of Pyruvate to Lactate compared to healthy tissues. To realize assessment of cancer metabolism via hyperpolarized 13C also in humans, our research group currently establishes this cutting-edge technology using a SPINlabTM DNP system for clinical application.
Publications of the group
Contact
-
Dr. Andreas Korzowski
Group leader
-
Dr. Philip Boyd
Project group leader
-
Dr. Vanessa Franke
Project group leader