7T MRI: RF Systems and Concepts
7T MRI: RF Systems and Concepts
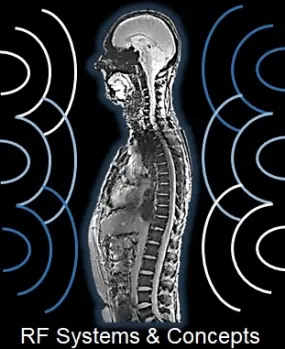
Our research group develops multichannel transmit systems for use in ultra-high field MRI systems to facilitate imaging of large volumes in the human body. We aim to provide tools that allow clinicians to make use of the full potential of ultra-high field MRI.
The work of the group can be divided into two parts: Hardware and Concepts.
On the hardware side, we work on the multi-channel transmit systems, including modulators, high power RF amplifiers, RF supervision hardware, transmit/receive switches, as well as RF arrays.
On the concepts side, we work on improving the use of the hardware, for example by introducing improved compression algorithms for the data fed into RF supervision hardware.
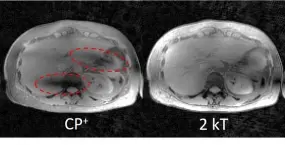
Since the beginning of Magnetic Resonance Imaging (MRI) there has been a constant drive to higher magnetic field strengths. The reason for this is the enhanced signal-to-noise-ratio (SNR) and improved contrast the increase in main magnetic field strengths provides. While 1.5T and 3T have become clinical standard, higher fields have emerged in scientific application with 7T having recently found its way into clinical practice.
While a rise in SNR and enhanced contrast can potentially increase the diagnostic value of the images acquired with higher field strengths, higher field strengths also bring challenges. The radio frequency (RF) fields used to excite the spins have to have the same frequency as the spins. This is called the Larmor frequency and it is proportional to the main magnetic field strength. Since the wavelength of RF waves is anti-proportional to frequency, the waves become much shorter at higher main magnetic field strengths. For example at 7T the wavelength in tissue at the Larmor frequency is about 11-13cm which is smaller than the diameter of even a human head. This leads to wave effects with brighter and darker areas with in the field of view, which severely affect image quality. To cope with this, multichannel approaches can be used where multi-channel transmit arrays are driven by the multiple amplifiers of a multi-channel RF system. With such a system, the effects of the reduced wavelength on the image quality can not only be reduced, but also more sophisticated transmit techniques like selective excitation can be enhanced. Furthermore, the increased degrees of freedom provided by multichannel transmit systems allow more control over local specific absorption rate (SAR), allowing for shorter repetition times and higher flip angles.
Our group has developed and implemented a 32 channel transmit system as an add-on for the 7T MRI system of the DKFZ [1].
The system consists of 32 IQ-modulators, 32 RF 2 kW peak power amplifiers with pre-distortion, a power supervision for patient safety, and integrated RF body array and peripheral devices to control correct timings.
The high channel count and high RF power of the system allow to acquire images with a large field of view tailored excitation to get closer to the full potential of 7T MRI.
Currently we are working on:
- Modulators with higher resolution and sampling rate
- More efficient amplifiers for high duty-cycle sequences
- A more modular design to allow for even higher channel counts
References: [1] Orzada, S., et al. (2019). "A 32-channel parallel transmit system add-on for 7T MRI." PLoS One 14(9): e0222452
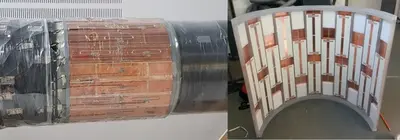
Radiofrequency (RF) arrays play a crucial role in MRI. In all modern MRI systems, arrays of RF coils are used to detect the signals emanating from the spins in the human body. Larger number of elements in such receive arrays can increase the SNR and allow for higher acceleration of the acquisition.
While clinical MRI systems at 1.5T and 3T use birdcage volume coils for transmission and arrays for reception, at ultra-high field (UHF) MRI, RF arrays are not only used for reception, but also for transmission. This is to gain more degrees of freedom when shaping the transmit field, as volume coils do not produce a homogenous excitation field at UHF. Similar to beam forming in modern radar technology, the elements of a transmit array in MRI are driven with individual amplitudes and phases and even different pulse shapes.
These arrays can be characterized be a multitude of criteria. They should have a high transmit efficiency to provide a strong excitation field with the transmit power provided by the system, as well as a high SAR efficiency to allow for a strong excitation field without damaging tissue due to excessive heating. Furthermore the elements of the array need to be well decoupled and their respective transmit profiles need to be distinct from one another to provide a high degree of freedom for shaping the transmit field.
While transmit RF arrays at UHF are almost exclusively local arrays placed directly on the body, our group aims at developing RF arrays that are integrated in the MRI system behind the inner cladding of the bore, just like the transmit volume coils of lower field MRIs [1].
Our current and future work in this area includes:
- Finding transmit antennas best suited for integrated arrays
- Developing very thin arrays to fit between boreliner and gradient coil of the most modern MRI systems
- Developing meta material solutions for improved coil performance
References: [1] Orzada, S., et al. (2019). "A 32-channel parallel transmit system add-on for 7T MRI." PLoS One 14(9): e0222452
Patient safety is an important factor in MRI. The RF power transmitted to excite the spins in the human body can heat up and potentially damage the tissues. To prevent this, the specific absorption rate (SAR) in W/kg is limited by guidelines and MR systems need to make sure to stay below the specified maximum values for whole body and 10g-averages at all times.
While SAR supervision of single channel systems is fairly easy as the SAR is just a linear function of the input power, the situation is more complicated in multi-channel transmit systems. Here, the SAR is a function of the complex excitation vector, containing the signal of all channels in amplitude and phase.
As the SAR in the human body cannot be measured, sophisticated simulations with exact coil models and high resolution human body models are performed. These simulations provide SAR matrices (S-matrices) which can be used to calculate the SAR by a simple vector-matrix-vector multiplication with the excitation vector. Unfortunately, there is an S-matrix for each 10g-averaging volume in the body which results in millions vector-matrix-vector multiplications to calculate the SAR. Since the excitation vector can change within a few µs, an online SAR supervision on an MRI has to do these calculations very quickly. Fortunately, it is possible to use compression algorithms which can trade the number of matrices for this calculation for an overestimation of the SAR [1-5]. This smaller set of matrices are called virtual observation point (VOPs).
Our recent investigations have shown that even with the best available compression algorithms, the computational burden for an SAR supervision increases with the number of channels to the power of more than 4 [6], making this computational burden the most difficult obstacle for increasing the number of RF channels in an MRI system.
Current and future work in this area includes:
- Investigating the field strength dependence of the number of VOPs
- Improving the speed of the SAR compression
- Finding improved post-processing algorithms of VOPs
References:
[1] Eichfelder, G. and M. Gebhardt (2011). "Local specific absorption rate control for parallel transmission by virtual observation points." Magn Reson Med 66(5): 1468-1476
[2] Lee, J., et al. (2012). "Local SAR in parallel transmission pulse design." Magn Reson Med 67(6): 1566-1578
[3] Orzada, S., et al. (2021). "Local SAR compression with overestimation control to reduce maximum relative SAR overestimation and improve multi-channel RF array performance." MAGMA 34(1): 153-163
[4] Orzada, S., et al. (2021). "Local SAR compression algorithm with improved compression, speed, and flexibility." Magn Reson Med 86(1): 561-568
[5] Orzada, S., et al. (2021). "Post-processing algorithms for specific absorption rate compression." Magn Reson Med 86(5): 2853-2861
[6] Orzada, S., et al. (2023). "An investigation into the dependence of virtual observation point-based specific absorption rate calculation complexity on number of channels." Magn Reson Med 89(1): 469-476
Publications of the group
Contact
-
Dr. Stephan Orzada
Group leader