Redox Regulation
- Cell and Tumor Biology

Prof. Dr. Tobias Dick
We aim to understand how tumor cells adapt to oxidative stress.
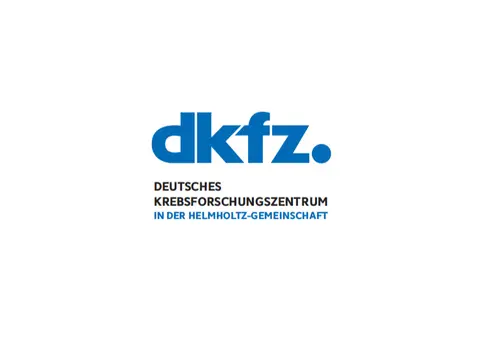
Our Research
Redox regulation is a process by which endogenous oxidants and reductants, derived from metabolism, dynamically modify proteins (including metabolic enzymes and transcription factors) to change their functional behavior in an adaptive manner. Research in recent years has started to reveal how tumor cells sense redox changes to trigger rapid adaptation and lasting cytoprotection.
Our group aims to understand the relevant sensing and signaling pathways, as well as the mechanisms of stress tolerance. Based on mechanistic insight, we develop tools enabling the visualization and manipulation of redox regulation inside living cells and model organisms. We employ these tools to better understand stress adaptation in both healthy and malignant situations. We are interested in intervention strategies that selectively disrupt cytoprotective mechanisms in malignant cells.
Key Publications
Marker T, Steimbach RR, Perez-Borrajero C, Luzarowski M, Hartmann E, Schleich S, Pastor-Flores D, Espinet E, Trumpp A, Teleman AA, Gräter F, Simon B, Miller AK, Dick TP
Eid M, Barayeu U, Sulková K, Aranda-Vallejo C, Dick TP
Talwar D, Miller CG, Grossmann J, Szyrwiel L, Schwecke T, Demichev V, Mikecin Drazic AM, Mayakonda A, Lutsik P, Veith C, Milsom MD, Müller-Decker K, Mülleder M, Ralser M, Dick TP
Pedre B, Talwar D, Barayeu U, Schilling D, Luzarowski M, Sokolowski M, Glatt S, Dick TP
Barayeu U, Schilling D, Eid M, Xavier da Silva TN, Schlicker L, Mitreska N, Zapp C, Gräter F, Miller AK, Kappl R, Schulze A, Friedmann Angeli JP, Dick TP
Talwar D, Messens J, Dick TP
Ezeriņa D, Takano Y, Hanaoka K, Urano Y, Dick TP
Stöcker S, Maurer M, Ruppert T, Dick TP
Morgan B, Van Laer K, Owusu T, Ezerina D, Pastor-Flores D, Amphonsah P, Tursch A, Dick TP
Peralta D, Bronowska AK, Morgan B, Dóka E, Van Laer K, Nagy P, Gräter F, Dick TP
Sobotta MC, Liou W, Stöcker S, Talwar D, Oehler M, Ruppert T, Scharf AN, Dick TP
Morgan B, Ezerina D, Amoako TN, Riemer J, Seedorf M, Dick TP
Albrecht SC, Barata AG, Grosshans J, Teleman AA, Dick TP
Gutscher M, Pauleau AL, Marty L, Brach T, Wabnitz GH, Samstag Y, Meyer AJ, Dick TP
Kienast A, Preuss M, Winkler M, Dick TP
Team
-
Prof. Dr. Tobias Dick
-
Ilona Braspenning-Wesch
-
Laura de Cubas Landaluce
-
Lukas Lang
-
Julia Langer
-
Alexander Pattberg
-
Danny Schilling
-
Nuriza Suleimenova
Open Positions
PhD Position
We are looking for a PhD student to join our efforts to understand the roles of biological sulfane sulfur species (persulfides, polysulfides) as antioxidants and as inhibitors of ferroptosis.
This work is part of the DFG-funded Priority Program SPP2306 "Ferroptosis: from Molecular Basics to Clinical Applications".
Highly motivated applicants with a solid background in chemistry/biochemistry/cell biology are encouraged to apply.
We expect excellent communication skills and enthusiasm for science.
Applications must include a cover letter, CV, copies of exams, and names of 2 referees.
Master Thesis Position
We are looking for a highly motivated student to work on a Master thesis in the field of free radical biology.
Get in touch with us
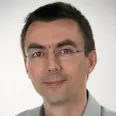